On a clear night in a remote area, if you were to look up, you might find a dense band of stars cutting across the dark sky. You’d be looking at a portion of our own galaxy, the Milky Way (MW). The MW is a collection of around 100 billion stars that are held together by gravity. How did our galaxy, and other galaxies like it, form? My work involves studying this very question, and the key to answering it lies in something called the galaxy’s stellar halo.
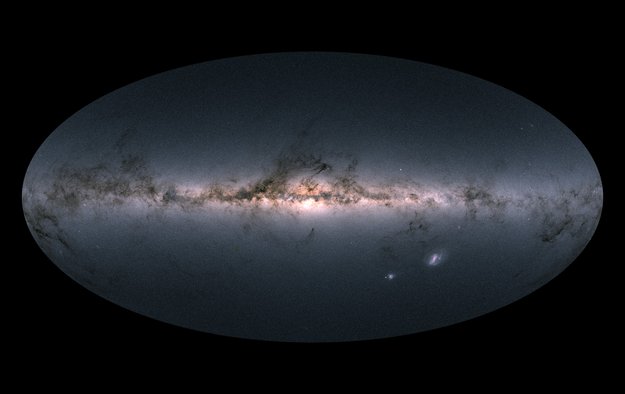
The Milky Way, viewed from our location in the Galaxy by the Gaia mission. Nearly 1.7 billion individual stars were used to make this map of the entire sky. Our location in the Milky Way enables us to study it in exquisite detail, but also presents complications.
ESA/Gaia/DPAC
Many galaxies in the universe are spiral galaxies like the MW, possessing a stellar disk and stellar halo. The disk is composed of stars in a plane that move in an orderly fashion. It makes up the part of the MW that you might see in the night sky. The stellar halo of a galaxy is made up of a faint collection of stars that orbit the center of the galaxy out to large distances. While the stars in the disk move uniformly, the stars in the halo move in a disorderly fashion, with a variety of speeds and directions. Although the stellar halo is not prominent to the naked eye like the disk is, it contains unique, invaluable information about how galaxies form.
The MW is just one of many similar 12 billion year old galaxies whose stellar halos are formed in the same way. The stellar halos of these galaxies are slowly built up by the remnants of smaller galaxies, which have hundreds to thousands fewer stars. These small galaxies are pulled apart by the strong gravity of the MW-like galaxy, and their stars become part of its stellar halo. Our current picture of how galaxies form on size scales of tens to thousands of light-years involves this hierarchical build-up of material.
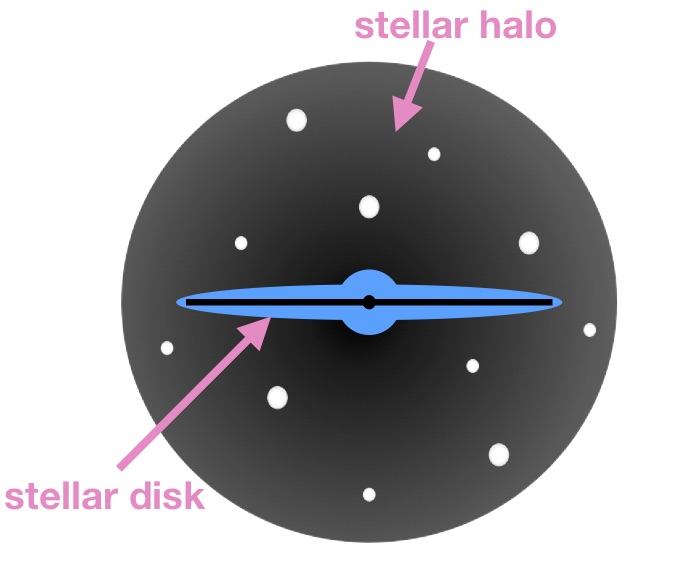
The structure of a MW-like or Andromeda-like galaxy. This type of galaxy has two main components made of stars, the stellar disk and the stellar halo. The disk is composed of stars in a plane that rotate in an orderly fashion. The stellar halo is approximately spherical in shape and contains stars moving in random orbits.
Ivanna Escala
Thus, studying the stars in stellar halos can tell us about the properties of the stars’ original galaxies; we can then use this information to more robustly test our models of galaxy formation. If the properties of these smaller galaxies disagree with the predictions from our models, then they update our understanding of the fundamental physics driving the formation of galaxies. For example, in the mid 1990s, astronomers thought that the MW’s stellar halo was built up by galaxies similar to its neighboring, small galaxies. Then they discovered that the chemical composition of the surviving small galaxies around the MW is very different from the chemical composition of the MW’s stellar halo. This was inconsistent with their models of galaxy formation; in response, they adjusted their models to account for the fact that the galaxies that built up the MW’s stellar halo are different than those that currently neighbor the MW.
Understanding a galaxy’s history through the chemical composition of its stars is like archaeologists reconstructing events in Earth’s past through buried remains of ancient civilizations. Astronomers use large ground-based telescopes as archaeological tools to observe individual stars in a galaxy. The light from a star in the stellar halo of a galaxy can be decomposed into a spectrum, which is the pattern of the star’s brightness with respect to the color of the light. A star’s light contains a wealth of information about its chemical composition, since each element imprints a unique signature in the spectrum. A dip in the star’s brightness at a specific color of light indicates the presence of a specific element. Each element is produced by a different astronomical event, so the elements we see in a star can tell us about what happened in the galaxy that the star originated from.
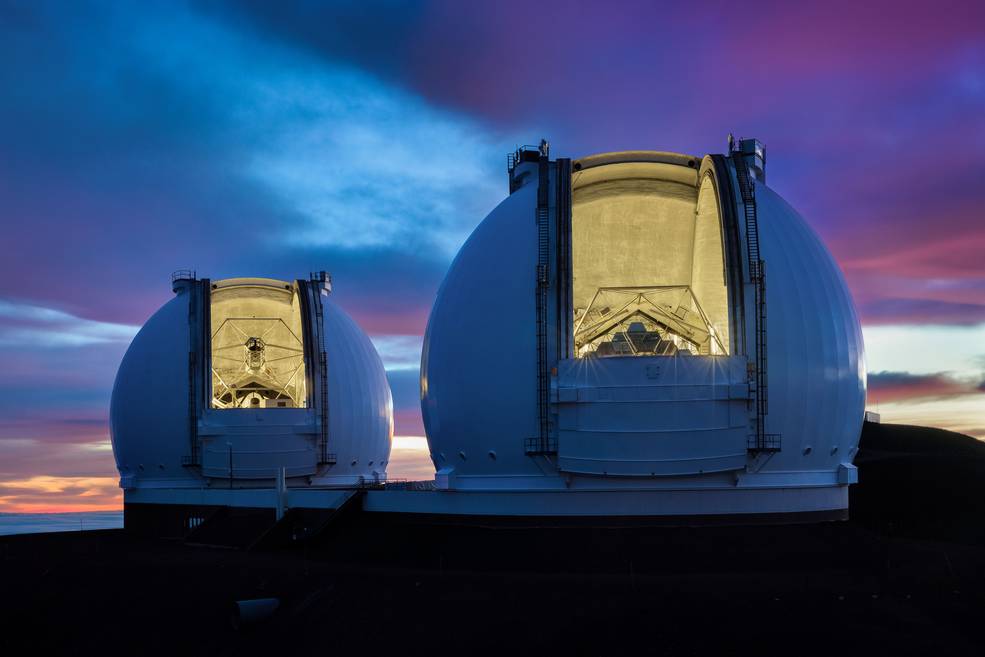
The Keck telescopes, located on the summit of Mauna Kea on the Big Island of Hawaii. These telescopes are some of the most powerful and scientifically productive large ground-based telescopes in the world. Such powerful telescopes in the Northern Hemisphere are necessary to measure the detailed chemical composition of stars in Andromeda.
Ethan Tweedie Photography/W. M. Keck Observatory, NASA
Two pieces of information that we can gather from stars in stellar halos are the history of star formation and the size of the galaxies they originated from. We measure star formation by looking at a group of elements called alpha-elements, which includes magnesium, silicon, and calcium. Alpha-elements trace the number of stellar explosions in a galaxy; in order for a star to explode, it obviously needs to have formed in the first place. If you know the amount of alpha-elements in a star from a stellar halo, you can learn how rapidly stars formed in the original smaller galaxies that now make up its stellar halo. So, if a star has a large amount of alpha-elements, the galaxy it came from had active star formation. In addition to alpha-elements, stars in stellar halos contain iron, a relatively heavy element compared to the alpha-elements. Astronomers use iron as a tracer of the total heavy element content of a star. Large galaxies are better at making heavy elements than small galaxies, so a star that was born in a larger galaxy will have more iron. Although iron cannot tell us whether the galaxy of origin was actively forming stars, it can tell us its likely size.
Astronomers have made measurements of alpha-elements and iron for hundreds of thousands of stars in the MW. As a consequence, most of our understanding of how galaxies form comes from the MW. If the MW turns out to be an abnormal galaxy, this is problematic for our broader understanding of galaxies. A further complication has to do with studying the chemical composition of stars in the MW while being located inside it. It’s like trying to figure out what your house is made of without being able to leave the couch in your living room. But you can look out your window at other houses – other galaxies – to get a pretty good idea. So which other galaxies, if any, can we study?
The Andromeda galaxy is ideal for studying how galaxies form because it is the closest galaxy of similar size to the MW. But Andromeda is still more than 2.5 million light-years away, or 130 billion times the distance between the Earth and the Sun. This large distance has historically posed a challenge to astronomers attempting to study it. However, there are still advantages to studying Andromeda. It is oriented nearly edge-on relative to us, such that we can obtain a spectacular view of its stellar halo.
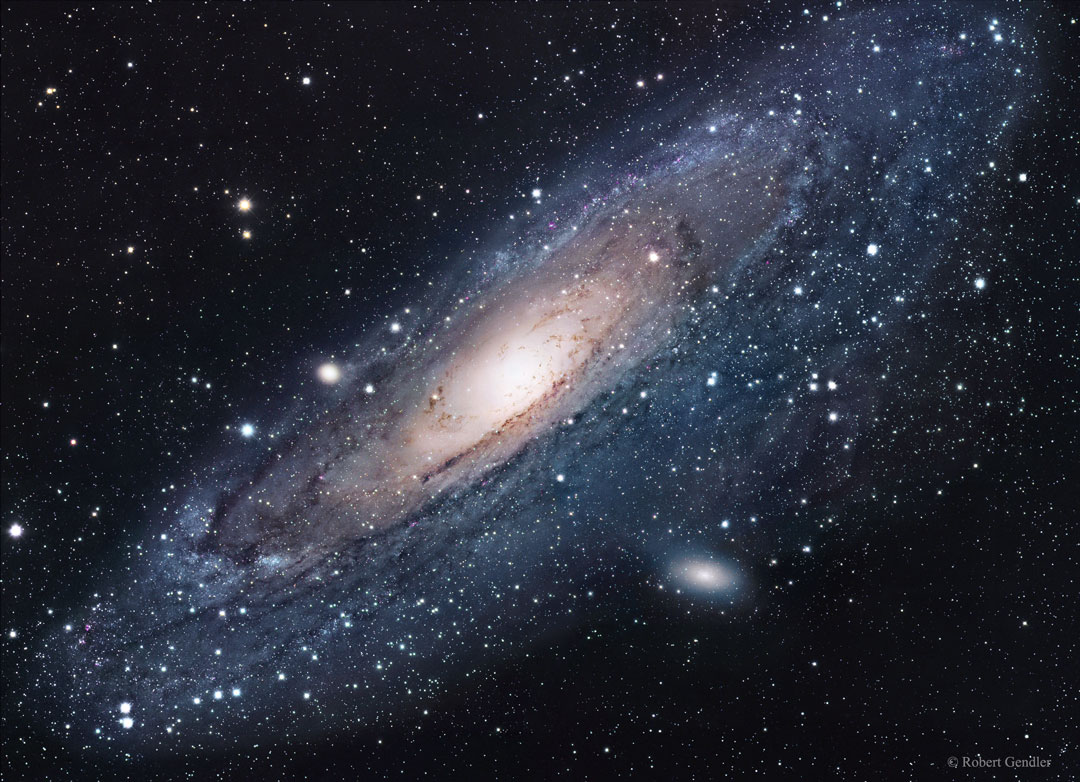
The Andromeda galaxy, the nearest large galaxy to our own Milky Way galaxy. The Milky Way is thought to look very similar to Andromeda. Andromeda is distant – about 2.5 million light-years away. Much is still unknown about Andromeda's history.
My research addresses the challenge of measuring alpha-elements and iron in stars in the stellar halo of Andromeda. Although our large ground-based telescopes are powerful, the great distance to Andromeda has prevented astronomers from making these measurements for more than a few stars in the past. I led a project to develop a new technique to improve the quality of our observations of stellar spectra in Andromeda by pushing the limits of what we can do with our existing technology. We leverage information from less detailed spectra that cover a broader range of the star’s light, rather than using more detailed spectra that cover a much narrower range of light; this actually results in a net increase in the quality of our measurement for stars in Andromeda. Using this technique, we can measure alpha-elements from the spectra of stars in the stellar halo of Andromeda for a large sample of stars for the first time. Until the next generation of large ground-based telescopes come online in the 2020s, the technique we have developed to improve the quality of our measurements with current telescopes is our best chance to learn about how Andromeda’s stellar halo formed.
As the first application of the new technique, I measured iron and alpha-element content for eleven stars in Andromeda’s stellar halo, significantly adding to the previous record of four stars. Although these eleven stars are located close to the center of Andromeda, at around 75,000 light-years away from its center, they contain far less iron than expected. This means that the region of Andromeda’s halo that I probed is deficient in heavy elements. Based on previous studies of the inner stellar halo of Andromeda, which used only approximations to measure the iron content, my collaborators and I expected the stars to be rich in heavy elements. It turns out that different parts of Andromeda’s stellar halo have different histories – that is, the original galaxies that were destroyed to make the halo are diverse.
These eleven stars belong to an ancient part of Andromeda’s stellar halo, assembled early in the universe. Their high alpha-element and low iron content suggests that the small galaxies of origin were young and actively forming new stars when they were pulled into the galactic halo. A similarly ancient part of the MW’s stellar halo is comparatively lower in alpha-elements, suggesting that Andromeda’s ancient stellar halo grew more quickly.
This is but one piece of the puzzle of Andromeda’s formation. In order to further understand how Andromeda formed, my collaborators and I have assembled more puzzle pieces by observing spectra of about two hundred stars in different parts of Andromeda’s stellar halo. Using the new technique, I have successfully measured the alpha-element and iron content for over sixty of these new stars. By collecting data on more and more stars in Andromeda, we can form a clearer, more detailed picture of the composition of the small galaxies that contributed to the entirety of its large stellar halo. Only then can we definitively answer whether the properties of these galaxies are shared by those that helped form the stellar halo of the MW. If our current models can account for the similarities and differences between these galaxies, then we will have more confidence in our understanding of the physics driving galaxy formation. If not, then we will have the opportunity to further our understanding of one of the most fundamental processes in the universe.
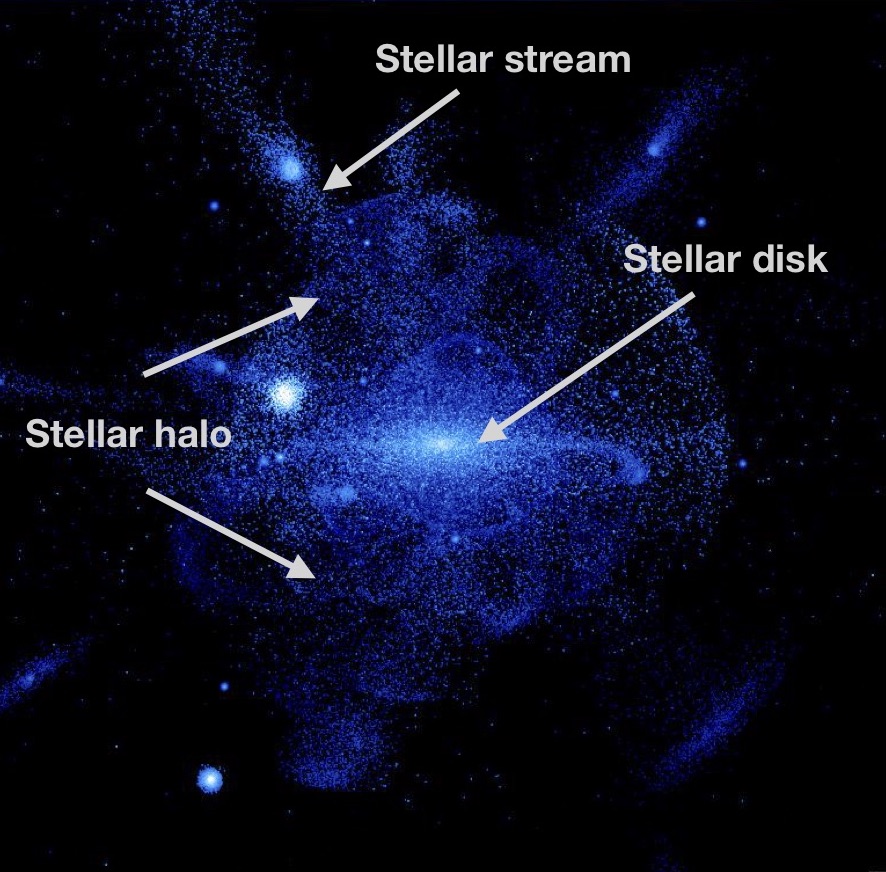
Stars in a simulated MW-like or Andromeda-like galaxy. The simulated galaxy is color-coded according to its brightness. The outskirts of this simulated galaxy is built entirely from small galaxies. Small galaxies in the process of being destroyed can be seen as thin streams of stars in the stellar halo.
Adapted from Bullock & Johnston 2005, The Astrophysical Journal, Volume 635, Issue 2, pp. 931-949
On a clear night in a remote area, set up your telescope and look past the Milky Way’s stars in the foreground, until you find Andromeda. To many, it will appear as nothing more than a fuzzy blob of light, but you’ll know that it’s a galaxy as vast and wondrous as ours. And despite the similarities to our own galaxy, you’ll know that its stellar halo tells us a unique story of its beginnings billions of years ago. The story of Andromeda provides us with a broader context for how galaxies form and grow, helping us understand our own galaxy’s place in the history of the universe.
Cover Image: Image Credit: NASA/ESA/Z. Levay (STScI)