It’s July, the heart of winter in the Southern Hemisphere. Antarctica, that last bastion of wilderness, is sheathed with a ring of sea ice. Scientists studying the Southern Ocean, the band of water encircling the frigid continent which acts as the conduit for waters passing between the Atlantic, Pacific, and Indian ocean basins, have long since left or are hunkered down on the land mass itself, enduring temperatures as low as -50˚C (-60˚F). Even the icebreakers, ships sturdy enough to break through meters of sea ice, that normally sail from some of the southernmost cities in the world—Punta Arenas, Chile; Cape Town, South Africa; and Christchurch, New Zealand—dare not brave the ferocious winds and swift currents associated with the Antarctic Circumpolar Current that are strongest in winter. Palmer Station, a U.S. research base supplied solely by ship, loses its only connection to the rest of the world from June to October each year. During (austral) winter, the waters in the Southern Ocean are all but unobserved … by humans.
On the 24th of July a lonely autonomous device, roughly 5 feet tall and cylindrical, pumps air into an external bladder and ascends to the surface of the ocean from 2 kilometers depth, taking measurements every few meters along the way. This floating device is one of a community of almost 4,000 across the world known as the Argo array. For most of their 3 to 5-year lifetimes, they drift around in the ocean following the prevailing currents at 1 km depth. Every 10 days, however, they dive to 2 kilometers depth and then ascend to the surface while taking measurements. At the surface, they relay water properties such as temperature, salt content, and oxygen concentration to data servers around the world, where remote software processes it into a form available for use and download from a central web server. At 64˚56’S and “only” 450 kilometers from the Antarctic coastline our lonely device is currently the southernmost active float in the world. It, along with its brothers and sisters in the Argo array, provides vital information on the waters of the Southern Ocean, including helping scientists track Circumpolar Deep Water, a warm, subsurface water mass implicated in melting ice shelves in Antarctica.
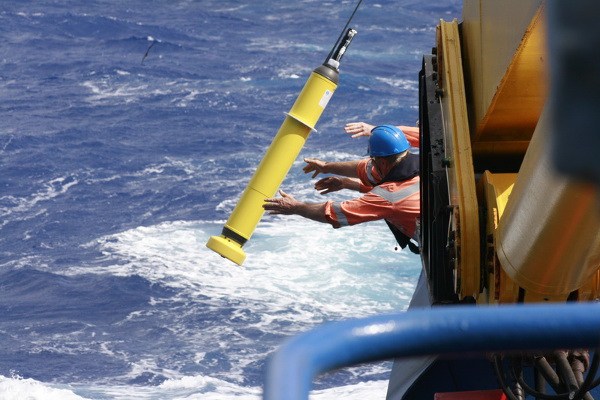
A marine technician deploys an autonomous Argo float into the Southern Ocean, where it will begin its journey through the ocean. This is the last human contact it will have for its expected lifetime of 4 years.
Alicia Navidad, CSIRO
The Argo array is but one of many autonomous systems centered on the scientific enterprise. By autonomous I mean that after the initial formulation, design, programming, and so forth, the large majority of a mission or platform’s time, data acquisition, and processing is done with minimal to no human involvement, in contrast to traditional research methods. While Argo is the largest and best-known autonomous mission in oceanography, the principle exists in most, if not all, scientific disciplines. Satellite observations, for example, are largely or primarily autonomous. For spacecraft far from earth, such as the recent New Horizons mission to Pluto, considerable autonomy is imperative due to connectivity issues. Much closer to home, even routine measurements, such as NMR for chemistry research, are increasingly being automated to at least some degree for a variety of academic and educational reasons.
Why is automation so important, and what makes it likely to play an even greater role in science in the future? There are five primary reasons: money, time, coverage, expertise, and data accessibility and reproducibility.
First, that all-important factor: money. We’ll again take oceanography as an example. The cost to operate a scientific research vessel varies widely, but even smaller, coastal ships cost the scientific investigator—and thus the funding agency—over $10,000/day. Research cruises in remote locations or needing specialized equipment (e.g. icebreakers) cost significantly more. By contrast, the total cost of a single Argo float—including data handling and project management—is approximately $30,000, with an average lifetime of 4 years. Deployment costs are often negligible, since the Argo network typically deploys instruments from so-called ships of opportunity, which are ships conducting other studies in the region anyway. The cost of one Argo float is comparable to the costs of operating a research vessel for a single day. Monetary limitations are even more important in other fields—the cost of sending a human to Mars is considerably higher than sending an autonomous rover, for example.
The second benefit is time. Simply traveling to a remote location in the ocean (to say nothing of Mars) can take weeks. Getting just one profile’s worth of oceanography data manually1 from a ship-based instrument takes hours. And while these things take just as long—or in many cases longer—when done autonomously, the number of person-hours is much, much lower. Autonomous observations mean less time used taking measurements and more time using the measurements to come to a better scientific understanding.
The third benefit, which somewhat follows from the previous two, is coverage. We’ve already run up to this before—automation enables us to study Mars via the rover program because it would cost too much to send humans, and to measure remote ocean locations because it takes a long time for scientists to get there. In polar oceanography, often the limiting factor is not (directly) time or money, but rather the availability of an icebreaking vessel or strong winds preventing a safe passage. Summertime measurements have been taken almost every year since 1993 between the southern tip of South America and Antarctica, across the treacherous Drake Passage, an especially important region because it allows for the passage of water from the Pacific into the Atlantic Ocean. Yet we have little information from that region during the winter—until last winter, that is, when two of Caltech’s own autonomous underwater vehicles braved the sea ice and winter storms to give an unprecedented look at wintertime ocean properties in this region.
Fourth, sometimes humans aren’t the most efficient at knowing where, when, how, or of what to make measurements. A prime example of this is the Burst Alert Robotic Telescope (BART) in Ondřejov, Czech Republic. BART looks at bursts of energy flung off from dying stars; observing and understanding these energy bursts helps explain the mechanisms that cause stars to explode. The trouble is that these bursts, which are distinct from, but easily mistaken for “common” supernovae, are unpredictable. Full characterization of this energy field requires recognizing the existence of a gamma ray burst soon enough (seconds-to-minutes) to turn other telescopes, like BART, to look at the same location and measure an “afterglow” effect from longer wavelengths. BART, which requires no human intervention during observations, is linked to the Gamma-ray Coordination Network (GCN), another autonomous network set up to monitor gamma ray burst discoveries. When a GCN alert comes, BART finds the target much faster than a human could. The entire sequence—from gamma ray burst detection from a telescope to image acquisition of the same location—requires no human activity. Not only is this a boon to astronomers who would rather spend their nights sleeping, but the faster response of computers which are pre-programmed to respond to events in real time enables much better measurements to be made and, ultimately, better science to be done.
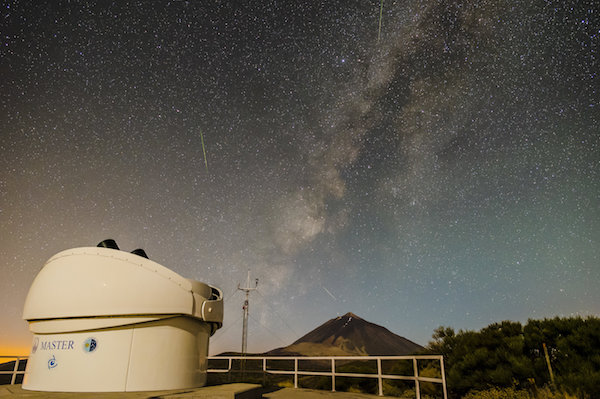
The MASTER-IAC telescope in the Canary Islands is part of an autonomous array of telescopes that scientists use to study gamma-ray bursts, similar to BART (described above).
Daniel Padrón, IAC
Finally, autonomous science leads to improved data accessibility and reproducibility. Writing software to properly acquire, process, and/or distribute data requires enumerating specific steps to be taken; computers cannot validate data simply because “it looks right.”2 These steps provide a path to precise reproduction of the data, and it is straightforward to program a computer to log all of its actions in a perfect “laboratory notebook.” In addition, data acquisition is often automated because of a large number of data streams; organizing this data into a common format is necessary for effective automated techniques, and has the pleasant side effect of, in general, providing a common and clear format for other scientists to access.
Of course, not all research can be autonomous. Often important discoveries are made because humans went “into the field”—whether on ships in the ocean, in landers to the moon, or into observatories during an all-night observing session—and experienced something they couldn’t have predicted. In 1903, the Swedish oceanographer Vagn Wilfrid Ekman discovered that friction caused by wind at the ocean surface moves the waters beneath at a 90° angle to the wind (the so-called Ekman Transport) only because of the travails of Fridtjof Nansen, a Norwegian whose expedition to reach the North Pole through sea ice drift was unsuccessful due in part to this effect. More recently, a massive plankton bloom was discovered underneath the ice in the Arctic—where the amount of available light was conventionally thought to be so low as to preclude any photosynthesis—thanks to a fortuitous find by a scientific research vessel in the area. That being said, for all of the reasons above, I predict that science of the future will rely much more heavily on autonomy—and will be the better for it.
Footnotes
1: ‘Manually’ in this case typically means being inside a ship on site while a suite of instruments is lowered from the ship into the water and autonomously takes measurements. I have had to do truly manual measurements – lowering an open canister to a given depth (defined by how much rope was let out) in the ocean and dropping a weight down the connecting rope to activate a mechanism manually closing the canister, trapping the water inside – and can confirm that it is a quite arduous process for a single measurement, especially in the frigid waters surrounding Antarctica!
2: In scientific publications, one can’t say simply that data “look right”, so this clause is typically replaced with something like “validated through visual inspection”.