United States Army Rocky Mountain Arsenal, Colorado, April 24, 1962 – The first of a decade-long series of earthquakes ruptures the ground miles beneath the state of Colorado. Seismometers and seismologists in Denver are equally shaken: Colorado is a relatively calm area, tectonically speaking.
This unusual seismic activity soon captures the attention of seismologist (and Reverend) Joseph V. Downey, who is among the first to infer a possible link between the earthquakes and a mile-deep high-pressure well put into operation earlier that year. The well is being used by a nearby chemical-manufacturing plant to dispose of large quantities of contaminated water—5 millions gallons per month, to be exact. A few years later, two studies1, 2 prove Downey’s suspicions right by confirming the existence of a correlation between the occurrence of earthquakes and the volume of injected fluids.
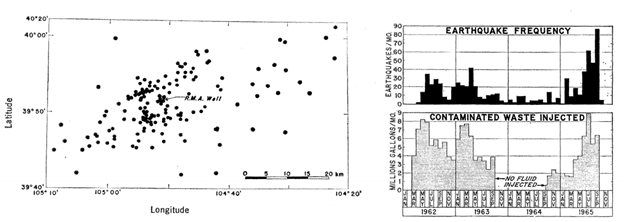
A 1968 publication showing the correlation between fluid injection and earthquakes at the Rocky Mountain Arsenal, Denver, Colorado. (Left) Location of wastewater disposal well and epicenters of earthquakes. (Right) Correlation between the number of earthquakes and the volume of fluid injected.
The Denver Earthquakes of the 1960s are a few of many examples of humans causing earthquakes. In fact, over the years, earthquakes have been attributed to human activities ranging from nuclear bomb detonation and dam impoundment to oil and gas extraction and underground mining.
The geo-energy industry is one such contributor to human-caused earthquakes. Geo-energy is a collective term for energy sources and technologies that come from or interact with the earth’s crust (i.e., the first 20 miles beneath our feet). Recently, the increasing frequency of earthquakes accompanying the rapid development of the industry has caused both concern and excitement in the earthquake science community. Unlike natural earthquakes, human-made earthquakes are not necessarily located in areas known to be tectonically active, like California and Japan. Thus, there is an implied risk of inducing damaging earthquakes in regions with little to no earthquake preparedness. On the other hand, earthquakes generated by the industry provide an unprecedented opportunity to unravel the deeply buried mysteries of earthquake physics.
Technologies used in the geo-energy industry often involve high-pressure injections into the earth’s crust. Enhanced geothermal systems, for example, involve increasing rock permeability (i.e., the capacity for fluid to flow through the rock) via high-pressure injections in order to access the naturally occurring geothermal energy at depth. Another example of geo-energy is carbon sequestration, which consists of injecting carbon dioxide into sealed but highly permeable rocks as a means of reducing the amount of carbon emitted to the atmosphere.
Probably the most familiar technology used in the industry is that of hydraulic fracturing, colloquially known as ‘fracking’, which is used to collect natural gas trapped in rocks. The technique consists of injecting a mixture of water and chemicals at high pressure into the ground, again with the goal of increasing the permeability of the rocks. Although this process facilitates pumping the natural gas back to the surface, it also generates large volumes of contaminated water. Because treating the used water is extremely expensive, the wastewater is instead disposed of in permeable rocks several miles beneath the earth’s surface.
In the central and eastern United States, seismicity is heavily correlated with the production of natural gas. There, the seismicity rate has increased from an average of 21 events per year to a shocking 150 events per year since 2009 (see below). 2009 also marks the beginning of a period of intense natural gas production in the US. Although the process of hydraulic fracturing itself can trigger earthquakes (as is happening in western Canada3), the majority of induced earthquakes in the region are in fact attributed to the associated wastewater disposal process4.
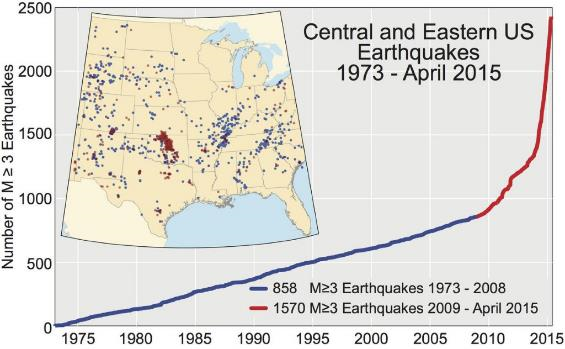
Earthquake count in central and eastern United States, 1973–2015.
The risk of damage is so tangible in some cases that new zones of high induced seismicity, such as Oklahoma, have been added to seismic hazard prediction maps (below). These maps are used by civil engineers to design earthquake-resistant structures and by insurance companies to quantify earthquake risks. To date, the highest magnitude earthquake known to have been induced by wastewater injection is a 5.9 event in Oklahoma—quite the event for a state where buildings are designed with little thought to earthquake safety. More worrying, the occurrence of earthquakes of even greater magnitudes may be possible5.
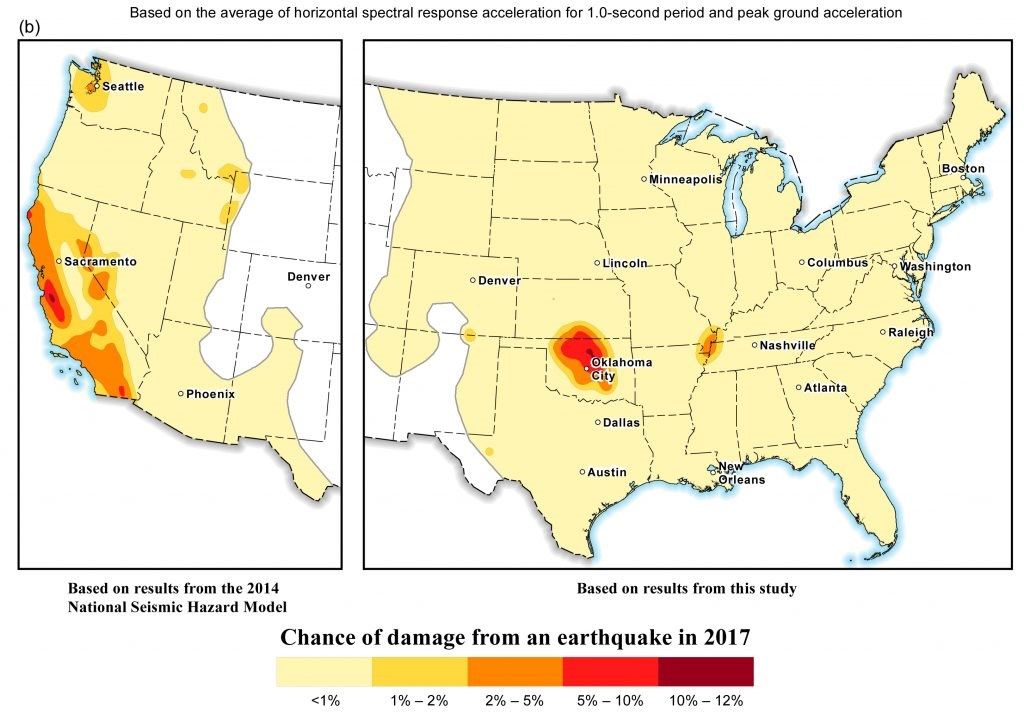
Forecasted chance of damage from an earthquake updated for induced earthquakes in central and eastern United States.
While human-caused earthquakes have the potential to cause significant damage, they also have the potential to help us understand the mechanics of natural earthquakes, which are generally much more destructive. To understand why that is, let us first review the basics of tectonic earthquake mechanics.
Most earthquakes occur on geological structures called faults. Faults are necessary to accommodate the relative motion between the ever-moving tectonic plates. For example, the famous San Andreas Fault, which crosses most of California, hosts the horizontal motion between the Pacific and North American Plates. As one can imagine, however, sliding two gigantic blocks of solid earth past one another is no easy task and often meets resistance. This resistance is the driving force that leads to earthquakes: when the force between the plates exceeds the strength of the fault, the fault starts to slip seismically and an earthquake occurs.
One simple explanation for fluid-induced anthropogenic earthquakes (those caused by humans) is that injecting fluids into the crust increases the ambient fluid pressure and unclamps, or loosens, the fault interface, thus making it easier for the fault to slip. While tectonic earthquakes are not caused by such fluid injections, naturally occurring fluids present in the crust have long been thought to play an important role in earthquake processes6.
Although natural and anthropogenic earthquakes do not have the same triggers, the fact that they occur in the same or similar environments makes us, earthquake physicists, believe that their mechanics are also similar. The major advantage of studying human-caused earthquakes over natural earthquakes is that we have far better control of where and when they will occur, thus allowing us to deploy seismometers and deformation sensors in the region of interest before the fact. Such measurements could provide a picture of what happens before, during and after an earthquake in a way that has previously never been captured. A team of seismologists are even planning a full-blown earthquake experiment in which fluids would be injected into a known fault structure and the resulting motions and perturbations captured with nearby monitoring wells7. Of course, this would be done in a remote location to minimize the risk of damage.
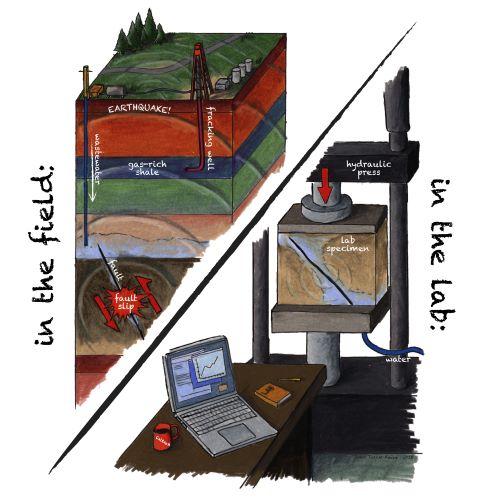
In the lab, earthquake physicists can compare real data to their simulations, which helps them develop better models to understand future earthquakes.
James Keane
For my part, I prefer to study the problem within the safe confines of computer modeling. As part of my Ph.D. research, I compare measurements from small field and laboratory injection experiments to our numerically modeled earthquakes. With such comparisons, we hope to understand which mechanisms play an important role in explaining the observed fault slip behavior. One question that we would especially like to answer is why fault slip, whether of human or natural origin, sometimes causes earthquakes and sometimes doesn’t, and whether fluids have anything to do with it.
This question has major implications for earthquake hazards— if we know what promotes slow, stable slip, we can one day hope to relieve the built up energy of major fault zones in a controlled, non-destructive manner thanks to fluid injections. Imagine a world in which we could control the release of energy stored in a fault, the way steam is slowly released from a pressure cooker. Imagine the destruction we could prevent. An earthquake physicist is allowed to dream…