Though people have been seeking their place in the universe and dreaming of life beyond the Earth since the beginning of human history, it is only recently that we have begun to apply the tools of science to interrogate these most fundamental mysteries. Astrobiology is the new science of life in a universal, rather than Earth-centric context, seeking to answer questions like what role life plays in changing planets, where we might expect life to exist beyond Earth, and how we could detect alien life within our solar system and beyond. One question, though, lies at the heart of every other in this field: how does life begin? In order to consider life throughout the universe, we need to understand the conditions and processes which drive life to emergence.
We know that life emerged from non-life at least once in the history of our planet, but it happened so long ago that piecing together an evidence-driven story for exactly how it happened is difficult. Until recently, few scientists put forward serious ideas describing the origin of life. In the past few decades, however, astrobiologists have made some amazing progress, and there is plenty we do know about how life got started even though researchers still disagree on some of the particulars. As it stands today, mainstream hypotheses tend to sort into one of two camps: genetics-first or metabolism-first. Researchers working on genetics-first hypotheses believe that genetic information — the instructions for making life work which are passed down parent to child — came about first, stored in molecules similar to modern DNA and RNA. If you’ve ever heard of the “RNA World”, you know something about this idea. Metabolism-first hypotheses describe a different origin story, one which begins with the chemical machinery life uses to extract energy from its environment. In other words, information-first ideas focus on what life is, while metabolism-first origins hinge on what life does. Each of these hypotheses have different implications for where life should be expected to exist in the universe and where we could go looking for it within our own solar system.
You’re probably already familiar with the most popular incarnation of the information-first story: the so-called “primordial soup theory.” This is the narrative for life’s earliest days that usually gets the spotlight on popular science programs like Cosmos. There’s good reason for the soup theory’s popularity – its earliest form was conceived by none other than Charles Darwin, who imagined life beginning in a “warm little pond with all sorts of ammonia and phosphoric salts, light, heat, electricity etcetera present” where proteins could form and undergo “still more complex changes” towards life. The famous Miller-Urey experiments, which involved electrocuting a gas mixture representing the primordial Earth’s atmosphere, proved that carbon-based molecules like those life uses, including the small molecules that link together to form DNA and amino acids, could be created naturally by lightning strikes on the early Earth. Waters deep in the past might well have contained the building blocks of life, both on Earth and elsewhere in the solar system: some scientists studying Mars believe that its northern hemisphere was once covered by an ocean. Depending on the kind of atmosphere which existed on Mars at the time, organics could have been created there in the same way as on Earth.
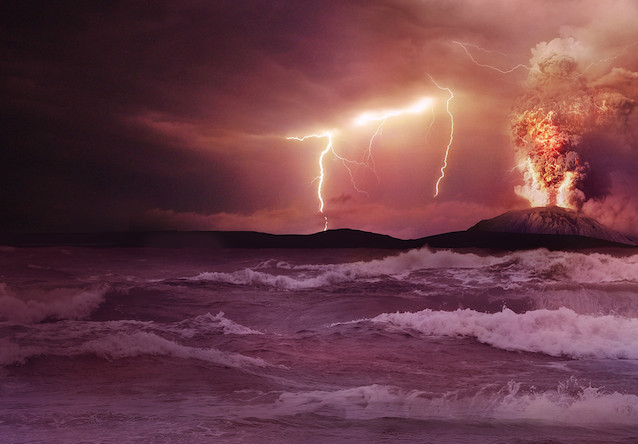
Artistic rendition of the early Earth. Lightning strikes are one proposed mechanism for the formation of the building blocks of life
NASA
Modern variations on the soup theory are numerous and diverse in their specifics. It’s somewhat outdated to believe that life began floating freely in the ocean – most recent iterations of the soup theory have embraced the “little” aspect of Darwin’s warm little pond, placing the emergence of life in a tide pool, geyser, hot spring, or volcanic hydrothermal field. The logic behind removing the origin of life from the ocean often comes down to concentration: the ocean is too big for organic compounds to condense enough to react and form life. Cycling wet and dry conditions in tidepool-like environments may have concentrated simple carbon compounds present naturally on the Earth, getting around this problem. The formation of lipid membranes (the “skins” which surround cells), which happens spontaneously, may also have played an important role as “bags” for keeping molecules close together as though they are in a cell. Other reasons scientists have begun to focus on environments like volcanic fields include the chemical composition of cells – these environments have chemistry more in line with the conditions we see inside cells today.
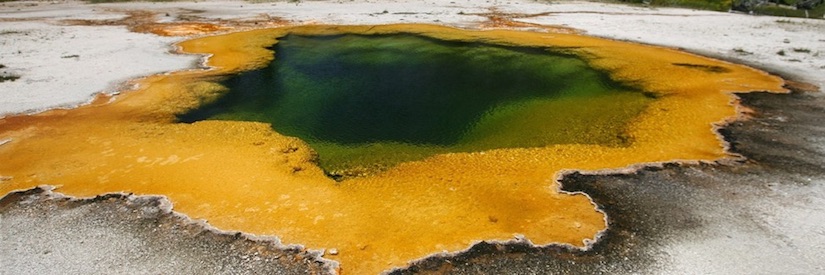
According to some modern variations on the soup hypothesis, life could have started on land, long ago at a hot spring not unlike this one at Yellowstone National Park. Moving the primordial soup from the ocean to an on-land spring or volcanic field address some of the weaknesses of the primordial soup theory by bringing in some of the important concepts from the metabolism-first story of the origin of life.
Jim Peaco, National Parks Service
In many ways, metabolism-first hypotheses came about as a response to flaws in the soup theory. Yes, the Miller-Urey experiment showed that it was possible to produce the building blocks of life under early-Earth conditions. However, even after decades sealed in their original flasks, the Miller-Urey mixtures failed to produce anything that could be called “alive”. Additionally, the soup theory can’t explain some of the chemical peculiarities common to all life, especially regarding how cells take in and store energy from their environments. One such chemical quirk related to cellular energy storage inspired Michael Russell, a researcher at NASA’s Jet Propulsion Laboratory (JPL), to suggest that life may have begun at alkaline hydrothermal vents before such vents were even discovered. Alkaline vents are rocky chimneys on the seafloor which spew out warm, low-pH, nutrient-rich water ions (charged molecules) generated by geochemical processes. The conditions which would have existed at these places in the distant past, when Earth’s ocean chemistry was different, share many similarities with the conditions cells create within themselves to generate and store energy. This has led researchers like Russell to believe that life could have begun at vents with the development of metabolic chemistry before anything like DNA or RNA existed.
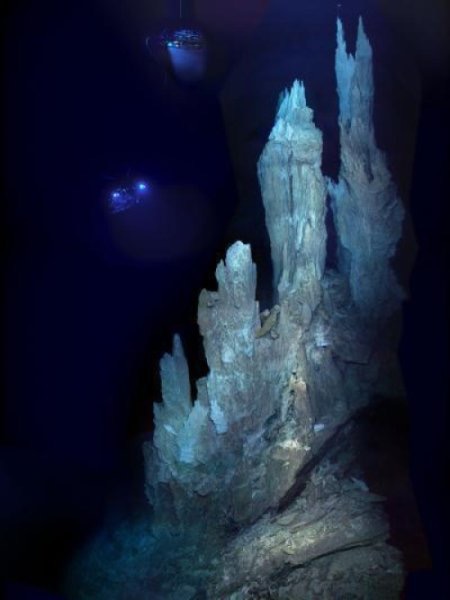
Although alkaline hydrothermal vents were predicted to exist by geochemists, they were only a theoretical possibility until 2000, when researchers from the University of Washington discovered the Lost City Hydrothermal Field in the Mid-Atlantic. Lost City is distinct from the previously discovered magma-fueled “black smoker” vents because its hot waters come from reactions between seawater and rocks at the seafloor. Billions of years ago, when ocean chemistry was different than it is today, sites like Lost City might have provided the perfect environment for the emergence of life.
Deborah Kelley, University of Washington, IFE, URI-IAO, NOAA
From an astrobiological perspective, these two groups hypotheses have some very important differences which result in different predictions for where we’d expect to find life in the universe. The hydrothermal vent hypothesis suggests that for life to emerge there need to be a specific chemical environment which isn’t present on all worlds. Continuing work on the geological history of Mars suggests that it probably lacked the right conditions for a hydrothermal-vent, metabolism-first origin of life. However, if the vent hypothesis is correct, we might expect to find life in the ice-covered oceans of Jupiter’s moon Europa and Saturn’s moon Enceladus. As researchers learn more about the chemistry of the waters on these worlds, astrobiologists will get a better idea as to whether or not a metabolism-first emergence could have happened there. Information-first hypotheses are a little more general, requiring only the synthesis of complex organics and reasonably habitable conditions for life to emerge. If this is how life gets started, we might expect to find life on any wet, rocky world with an atmosphere capable of producing lightning, or even in more exotic places like Saturn’s moon Titan — a world shrouded in a thick haze of organic molecules, with a surface temperature so cold that methane (natural gas) exists there as a liquid.
Both the metabolism-first and information-first camps continue to develop and change. In response to the development of the metabolism-first alkaline hydrothermal vent hypothesis, some researchers studying information-first origins have looked for new locations for their “warm little pools” which share some important characteristics with modern life’s chemistry. Volcanic pools and geysers have received substantial of attention in recent years, since the concentrations of certain ions in such places more closely mirror those in cell cytoplasm than those in ocean water. People studying hydrothermal vents as a possible origin site are now working on ways to get from metabolism to cell – how life could have left hydrothermal vents.
The origin of life will certainly continue to be a subject of intense debate among scientists for the foreseeable future, but that doesn’t mean that scientists aren’t making progress. And as we learn more about the early history of life on our own planet, we are better armed as astrobiologists to consider where and how often we might expect to find life elsewhere in the universe. By discovering our own origins, we might take the first step towards answering that most famous question: are we alone?